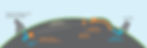
Fermilab is America’s leading particle physics laboratory. There is no doubt that the laboratory has a large and robust neutrino research effort and there is also no denying that the laboratory’s long term and flagship experiment is called DUNE, short for Deep Underground Neutrino Experiment.
In what might be a case of stating the very obvious, the DUNE detector will be located deep underground and will search for neutrino interactions. That sounds obvious enough. but, when you dig into the details, the reality is a bit more complex. DUNE actually consists of a couple of detectors: a small one located on the Fermilab campus and a much larger one located 800 miles away in Lead, South Dakota.
Fermilab will shoot the world’s most intense beams of neutrinos and antimatter neutrinos through both detectors to better understand a fascinating behaviour called neutrino oscillation. One of the key goals is to see if neutrinos and antimatter neutrinos oscillate identically.
If they don’t, this will be an absolutely crucial discovery that will help explain why the universe exists as we know it.
What are Neutrinos
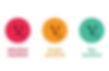
Neutrinos are the ghosts of the subatomic world and are very hard to detect. Because they experience only the weak nuclear force, they can easily pass through matter. For instance, neutrinos can pass through the entire Earth, with only a very tiny probability of interacting. In fact, that happens all of the time with neutrinos produced in the Sun. There are three different types of neutrinos, each denoted by the Greek letter and a subscript that identifies which kind they are.
The three types are the electron-type, the muon-type, and the tau-type. Each type has a special affinity for a specifically charged lepton like the electron-type neutrino is produced at the same time as an electron and so on.
These three types of neutrinos are similar, but different- like a cat, a jaguar, and a
Tiger. Neutrino oscillation is the phenomenon where neutrinos change their identity going from one to another and back again. It’s like a cat morphing into a jaguar and then a tiger and then back to a cat.
What will the DUNE experiment tell us?
Researchers have suspected neutrinos oscillated since the early 1970s and data recorded in the late 1990s proved that it’s true. We’ve spent the last two decades studying the phenomenon in detail. The DUNE experiment is designed to study neutrino oscillation and compare it to anti-neutrino oscillation.
A beam of nearly pure muon neutrinos or antineutrinos will be shot from Fermilab to western South Dakota. The detector on the Fermilab campus will characterize the beam when it leaves the site and the distant detector will study it when it arrives, 800 miles away.
These two measurements will allow researchers to determine how much oscillation occurred
while the particles were in flight. So, oscillation measurements like those are the bread and butter of the DUNE research program, but it’s not the driving point of the experiment. There are a couple of topics that are worth calling out.
Matter-antimatter symmetry
The first one has to deal with a very serious mystery of modern physics, specifically the seemingly silly question of why our universe is made of matter. That question just seems very peculiar- I mean- of course, the universe is made of matter. But it turns out that that ‘of course’ isn’t so obvious.
For instance, we know of a substance called antimatter which is a cousin of matter. Take matter and antimatter and combine them and they convert into energy. The converse is also true. Energy can convert into matter and antimatter. You can combine that observation with the theory of the Big Bang and you encounter a mystery.
The Big Bang says that the universe was once smaller and hotter and full of energy. As the universe expanded and cooled, that energy should have turned into equal amounts of matter and antimatter. And yet, our universe consists entirely of matter. So where did the antimatter go? We don’t know the answer to that, but we believe that there was a very tiny imbalance in the early universe, so that for every three billion antimatter particles, there were three billion and one matter particle. The three billion cancelled each other out and the one leftover is what made us.
I said that we don’t know how that happens, but we have found some small indications that the universe slightly favours matter over antimatter, but not enough to explain the mystery.
So that’s where neutrinos and the DUNE experiment comes in.
The DUNE experiment will study neutrino oscillation using both neutrinos and antimatter neutrinos. If we find that they oscillate to a different degree, that could be the crucial clue that explains, well, the whole mystery. Or not. That’s why we do the experiment- to help us find out if this line of thinking is true. So this study of matter and antimatter neutrinos is the biggest reason to build the DUNE detector, but there are a couple of others.
Proton decay
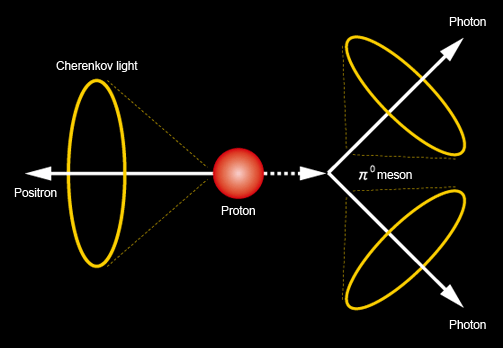
Another reason is that there are theories that say that ordinary protons will decay. Now our best theory of physics says that protons are stable and we’ve measured their lifetime and determined that if they decay they live for ten to the thirty-fourth power years. That’s ten billion, trillion, trillion years- which is enormously longer than the measly 14 billion year lifetime of the universe.
The DUNE experiment will be able to achieve comparable performance to the best-existing
measurements. And, for certain decays, it will be able to do better than any other detector.
And, if DUNE actually observes proton decay, that will completely rewrite the textbooks.
Detection of emitted neutrinos
There is a third neat thing that DUNE can do and that is to look for neutrinos emitted from a supernova, which is the explosion of a star. Using different apparatus, we’ve seen one such example before. In 1987, a star blew up in the Larger Magellanic Cloud, which is a small galaxy orbiting the Milky Way.
The biggest detector at the time, called Kamiokande II, saw a grand total of 12 neutrinos.
If you combine the neutrinos from all detectors that were active at the time, a total of 25
were seen. If the DUNE experiment were running back in 1987, it would have measured 120 neutrinos by itself. Such a measurement would help us better understand the dynamics of just what happens when a star collapses.
Supernovae are pretty rare, with only about one per galaxy per century, and the DUNE detector will only see supernovae in the galaxies closer than Andromeda, so DUNE researchers will have to get lucky to see one. But since the detector is being built anyway, it would be foolish to not use it to do some astrophysics as well.
Its sociological and technological impact
We’ve talked about why DUNE is very interesting scientifically, but it's also interesting
both technologically and sociologically.
The detector in Lead, South Dakota will be buried nearly a mile under the Earth’s surface in the Sanford Underground Research Facility. The detector will consist of almost seventy thousand tons of liquid argon, housed in four gigantic caverns. Each cavern will contain a detector 45 feet square and 200 feet long. Liquid argon was chosen as a technology because it allows researchers to record in detail the particles originating in collisions of neutrinos with atoms in the detector.
And we know that approach works because a prototype version of DUNE has been built and tested. The prototype is called ProtoDUNE and researchers have already used ProtoDUNE to look at particles passing through the detector.
DUNE is an international collaboration, involving researchers at institutions from 32 countries and CERN. CERN isn’t a country, but as the world’s other powerhouse particle physics institution, it gets a special mention. The DUNE collaboration really does have some of the world’s foremost neutrino experimenters among its collaborators.
So what’s the status of DUNE, you might ask? Well, it’s being built. The first of the caverns should have an operational detector by the mid-2020s. The others will follow shortly thereafter. I know that sounds like quite a ways away, but it’s not a lot of time to build a world-class beamline and facility. We’ll be buried in neutrino data before you know it.
DUNE is going to dominate the neutrino landscape for decades, resulting in fascinating physics. And that makes it very important because, as you know, physics is everything.